Pre-clinical Research & Development
Assay Development Support
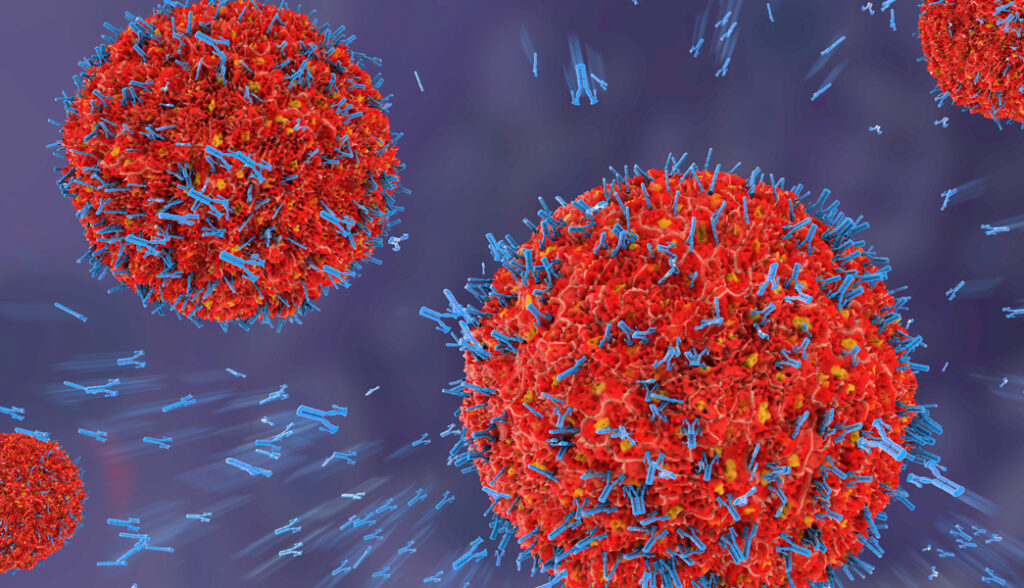
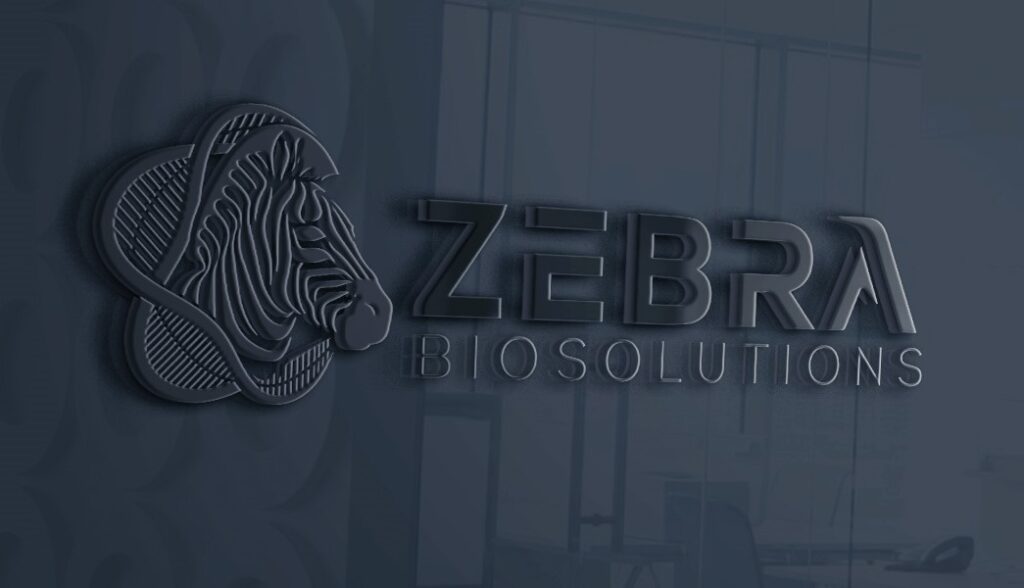
Our assay development program involves designing and optimizing task-dependent experimental procedures to measure a specific biological or biochemical activity. These assays are essential in various fields, such as drug discovery, diagnostics, and basic research. There are several approaches and methodologies for assay development, depending on the target, readout, sensitivity, and other factors:
Cell-Based Assays:
Functional Assays: Measure the function or activity of a target within the cell, often using reporters like luciferase or fluorescent proteins.
Cytotoxicity and Proliferation Assays: Determine the effects of compounds on cell viability or growth.
High-Content Screening (HCS): Utilizes automated microscopy to analyze cellular phenotypes.
Biochemical Assays:
Enzyme-Linked Assays: These assays measure the activity of enzymes. Examples include ELISA (Enzyme-Linked Immunosorbent Assay) for detecting specific proteins and Kinase Assays for enzyme activity.
Protein-Protein Interaction Assays: Techniques like Co-Immunoprecipitation and pull-down assays to study protein interactions.
DNA/RNA Interaction Assays: EMSA (Electrophoretic Mobility Shift Assay) and other techniques to study nucleic acid interactions.
Immunoassays:
Western Blotting: Identifying specific proteins using antibodies.
Flow Cytometry: Assessing cell populations and markers using fluorescent antibodies.
Utilizing antibodies to detect proteins in tissue sections or cells.
Receptor-Ligand Binding Assays:
Radio-Ligand Binding Assays: Measure the binding of labeled ligands to receptors.
Surface Plasmon Resonance (SPR): Assessing binding interactions in real-time.
Genomic and Transcriptomic Assays:
qPCR and RT-qPCR: Quantitative PCR methods to measure DNA or RNA levels.
RNA-Seq: Analyzing entire transcriptomes using next-generation sequencing.
Phenotypic Assays:
Whole Organism Screening: Using model organisms like zebrafish or C. elegans to assess compound effects on physiology or development.
Behavioral Assays: Assessing behavior in model organisms as a readout for neural or physiological function.
High Throughput Screening (HTS) and Automation:
Miniaturization: Developing assays in a smaller scale (e.g., 384 or 1536 well plates) to facilitate large-scale screening.
Robotic Automation: Automating pipetting and other processes to handle large compound libraries.
Biosensor and Diagnostic Assays:
Lateral Flow Assays: Rapid diagnostic tests like pregnancy tests.
Microfluidic Devices: Utilizing small volumes and channels to perform assays on chips.
In Vivo Assays:
Animal Models: Developing disease models in animals to evaluate drug efficacy or toxicity.
Clinical Trials: Assay development for monitoring patient responses, biomarkers, and clinical endpoints.
In Vivo Assays:
Animal Models: Developing disease models in animals to evaluate drug efficacy or toxicity.
Clinical Trials: Assay development for monitoring patient responses, biomarkers, and clinical endpoints.
In Silico and Computational Approaches:
Molecular Modeling and Docking: Virtual screening to predict ligand binding.
Machine Learning and AI: Utilizing computational algorithms to predict compound activity or toxicity.
In Silico and Computational Approaches:
Molecular Modeling and Docking: Virtual screening to predict ligand binding.
Machine Learning and AI: Utilizing computational algorithms to predict compound activity or toxicity.
In developing any assay, a variety of factors must be considered, such as sensitivity, specificity, throughput, cost, and robustness. Thorough validation, including the use of controls and understanding potential artifacts or interferences, is also critical to the successful development of an assay.
CAR-T, CAR-NK, and CAR-M Cell Technologies in Cancer Research
CAR-T, CAR-NK, and CAR-M Cell Technologies in Cancer Research
Chimeric Antigen Receptor (CAR) technology represents a significant advancement in the field of cancer immunotherapy. By modifying immune cells to express CARs, researchers have developed targeted treatments for various types of cancer. Let's explore CAR-T cells, CAR-NK cells, and CAR-Macrophages (CAR-M) and how they contribute to biomedical research.
CAR-T Cell Therapy:
Structure and Design:
CAR-T cells are T lymphocytes genetically engineered to express a chimeric antigen receptor (CAR) on their surface.
A CAR is composed of an extracellular domain recognizing a specific antigen, often a single-chain variable fragment (scFv), linked to transmembrane and intracellular signaling domains (e.g., CD3ζ
Application in Cancer Treatment:
CAR-T cells are designed to target specific cancer antigens, such as CD19 in B-cell malignancies.
Once the CAR-T cells recognize the target, they become activated, proliferate, and kill the cancer cells.
FDA-approved CAR-T cell therapies include Kymriah and Yescarta, for treating certain types of lymphomas and leukemias.
Challenges and Considerations:
CAR-T cell therapies can lead to severe side effects, including cytokine release syndrome (CRS) and neurotoxicity.
There are ongoing efforts to improve CAR-T cell safety, specificity, and effectiveness, including the development of "off-the-shelf" allogeneic CAR-T cells.
CAR-T Cell Therapy:
Structure and Design:
CAR-T cells are T lymphocytes genetically engineered to express a chimeric antigen receptor (CAR) on their surface.
A CAR is composed of an extracellular domain recognizing a specific antigen, often a single-chain variable fragment (scFv), linked to transmembrane and intracellular signaling domains (e.g., CD3ζ)
Application in Cancer Treatment:
CAR-T cells are designed to target specific cancer antigens, such as CD19 in B-cell malignancies.
Once the CAR-T cells recognize the target, they become activated, proliferate, and kill the cancer cells.
FDA-approved CAR-T cell therapies include Kymriah and Yescarta, for treating certain types of lymphomas and leukemias.
Challenges and Considerations:
CAR-T cell therapies can lead to severe side effects, including cytokine release syndrome (CRS) and neurotoxicity.
There are ongoing efforts to improve CAR-T cell safety, specificity, and effectiveness, including the development of "off-the-shelf" allogeneic CAR-T cells.
CAR-NK Cell Therapy:
Structure and Design:
CAR-NK cells involve natural killer (NK) cells expressing CARs. NK cells are part of the innate immune system, possessing intrinsic cytotoxic ability.
Similar to CAR-T cells, CARs in CAR-NK cells have antigen recognition and signaling domains.
Application in Cancer Treatment:
CAR-NK cells combine the specificity of CARs with the natural ability of NK cells to target cancer, offering a potentially less toxic and more controllable approach.
CAR-NK cells have been developed to target antigens like CD19 and HER2.
Challenges and Considerations:
CAR-NK cells may offer a safer profile, with less risk of CRS or graft-versus-host disease (GVHD).
Scaling up and ensuring consistent quality of CAR-NK cells can be challenging. Our scientists have developed innovative assays that mitigate these challenges and ensure batch-to-batch consistency and potency
CAR-Macrophage (CAR-M) Therapy:
Structure and Design:
CAR-M therapy involves modifying macrophages to express CARs.
Macrophages are immune cells involved in phagocytosis and antigen presentation.
Application in Cancer Treatment:
CAR-M technology aims to reprogram macrophages to recognize and phagocytose cancer cells.
By harnessing the natural ability of macrophages to engulf cells, CAR-M could provide a novel mechanism of action against tumors.
Challenges and Considerations:
CAR-M technology is relatively new and still in the early stages of development.
Designing CAR-M to balance anti-tumor activity without excessive inflammation or off-target effects is a key challenge.
CAR-based immunotherapies represent a powerful tool in the fight against cancer, with CAR-T cell therapy being the most advanced and clinically validated approach. CAR-NK cells offer potential advantages in safety and broader applicability, while CAR-Macrophages present a novel concept that is still being explored. Ongoing research is focused on enhancing efficacy, minimizing side effects, and expanding the range of treatable conditions. Additionally, combinations of CAR-modified cells with other therapeutic strategies are an area of active investigation.
Gene Therapy Research Support Services
Zebra BioSolutions Gene Therapy support solutions include the following:
Structure and Design:
Customized design, construction, and production of viral vectors such as AAV, lentivirus, and adenovirus.
Development of non-viral vectors, including nanoparticles and liposomes.
Pre-clinical Model Development:
Generation of in vitro and in vivo models for efficacy testing, including specific disease models.
Custom animal model generation using CRISPR/Cas9 and other gene editing tools.
Assay Development and Validation:
Design of in vitro assays for potency, specificity, and toxicity assessment.
Validation according to industry and regulatory standards.
Toxicology and Safety Analysis:
Conducting pre-clinical safety evaluations, including biodistribution studies.
Analysis of potential off-target effects and genotoxicity.
Scale-Up and Manufacturing Support:
Technical support for the scaling up of vector production.
Process development and optimization for manufacturing under Good Manufacturing Practices (GMP) conditions.
Regulatory and Clinical Trial Support:
Assistance with IND (Investigational New Drug) filings, including pre-IND meetings.
Support for the design of clinical trials, including phase I-III studies.
Bioinformatics and Data Analysis:
Genome-wide off-target analysis using next-generation sequencing data.
Bioinformatic pipeline development for analyzing gene expression patterns.
Cell Line Development and Authentication:
Development of stable cell lines for gene therapy applications.
Cell line authentication and mycoplasma testing.
Training and Consultation:
Custom training programs for biotech teams on the latest gene therapy technologies.
Consultation services to guide strategic decisions and troubleshoot challenges.
Intellectual Property (IP) Assistance:
Guidance on patent landscape, filings, and freedom-to-operate analyses in the realm of gene therapy.
Development of Protein Biologics and Antigens
Plasmid Design for Gene Expression:
Insert Selection:
Choose the gene or DNA sequence of interest.
Include elements like promoters, enhancers, and terminators to control the expression of the gene.
Vector Selection:
Select a suitable plasmid vector that can replicate in the host organism.
Consider elements like origin of replication, antibiotic resistance genes for selection, and any specific tags or markers needed.
Verification:
Confirm the correct insertion using techniques like sequencing, restriction analysis, or PCR.
Cloning Strategy:
Determine the restriction sites for cloning.
Utilize PCR, restriction enzymes, and ligation or other methods like Gibson Assembly to insert the gene into the plasmid.
Viral Delivery:
Viral Vector Selection:
Common viral vectors include lentivirus, adenovirus, and adeno-associated virus (AAV).
Consider factors like host range, integration, and safety.
Viral Packaging:
Incorporate the plasmid into viral particles in packaging cell lines. This may require additional helper plasmids or packaging proteins.
Safety Considerations:
Care must be taken to prevent replication-competent viruses and to handle viruses safely in the laboratory.
Viral Transduction:
Use the viral particles to infect the target cells.
The viral machinery facilitates the integration of the plasmid or expression cassette into the host genome, or episomal maintenance.
Non-Viral Delivery:
Physical Methods:
Electroporation: Applying an electric field to create temporary pores in the cell membrane.
Microinjection: Directly injecting the plasmid into the cell.
Gene Gun: Using high pressure to shoot DNA-coated particles into cells.
Chemical Methods:
Lipofection: Mixing plasmids with lipids to form liposomes that fuse with the cell membrane.
Calcium Phosphate Transfection: Precipitating DNA with calcium phosphate, which is then taken up by cells.
Polymer-based Transfection: Using cationic polymers to form complexes with DNA, facilitating uptake.
Biological Methods:
Bacterial Conjugation: Utilizing bacteria to transfer plasmids to other cells.
Conclusion:
CAR-based immunotherapies represent a powerful tool in the fight against cancer, with CAR-T cell therapy being the most advanced and clinically validated approach. CAR-NK cells offer potential advantages in safety and broader applicability, while CAR-Macrophages present a novel concept that is still being explored. Ongoing research is focused on enhancing efficacy, minimizing side effects, and expanding the range of treatable conditions. Additionally, combinations of CAR-modified cells with other therapeutic strategies are an area of active investigation.
Protein Expression
Protein expression and purification are fundamental techniques in molecular biology, biochemistry, and biotechnology, allowing researchers to produce and isolate specific proteins in relatively large amounts for further analysis.
Protein expression refers to the method by which proteins are synthesized, modified, and regulated in living organisms. In a laboratory context, it often refers to the production of a specific protein using recombinant DNA techniques. Here's a basic overview:
Host Selection: Various hosts can be used to express proteins, including bacteria (e.g., E. coli), yeast, insect cells, mammalian cells, and plant cells.
Transformation/Transfection: The expression vector is introduced into the chosen host. This process is called transformation for bacterial cells and transfection for eukaryotic cells.
Harvest: After allowing the host cells to grow and express the protein, the cells are harvested, typically by centrifugation.
Vector Selection: A piece of DNA that contains the gene of interest (e.g., the gene coding for the desired protein) is inserted into an expression vector suitable for the host.
Induction: Many expression systems have an induction step that triggers the production (or expression) of the protein of interest. For example, in many E. coli systems, IPTG is added as an inducer.
Protein Purification:
Once the protein is expressed, it often exists in a complex mixture with numerous other proteins, lipids, nucleic acids, and small molecules. To study the protein in detail, it's essential to isolate or "purify" it from this mixture. Here's a general process:
Cell Lysis: The harvested cells are broken open to release their contents. This can be achieved mechanically (e.g., by sonication or French press) or enzymatically (e.g., by lysozyme treatment for bacterial cells).
Chromatographic Techniques: These techniques separate proteins based on different properties:
- Affinity Chromatography: If the protein of interest is tagged (e.g., with a His-tag), it can bind to a specific resin and be selectively eluted.
- Ion Exchange Chromatography: Proteins are separated based on their charge using cationic or anionic resins.
- Size Exclusion Chromatography (Gel Filtration): Proteins are separated based on their size.
- Hydrophobic Interaction Chromatography: Proteins are separated based on their hydrophobicity.
Primary Recovery: Large cellular debris is removed, typically by low-speed centrifugation.
Protein Solubilization: Depending on the location of the expressed protein (e.g., cytoplasmic, periplasmic, membrane-bound), additional steps might be required to solubilize or extract the protein from cell fractions.
Concentration: Methods like ultrafiltration can be used to concentrate the purified protein.
Assessment: After purification, the quality and quantity of the purified protein are typically assessed using techniques like SDS-PAGE, Western blotting, and spectrophotometry.
Storage: Purified proteins can be stored for longer durations at low temperatures or in the presence of cryopreservatives like glycerol.
Other Considerations:
Tagged vs. Untagged: Some proteins are engineered with tags that aid in their purification (e.g., His-tag, GST-tag). After purification, these tags can be removed by specific proteases if needed.
Protein Refolding: If the expressed protein is found in insoluble aggregates (inclusion bodies), it might need to be solubilized in strong denaturants (e.g., urea) and then refolded into its native conformation.
Optimizing Expression: Sometimes, the initial protein expression might be low, or the protein might be insoluble. Optimization strategies might involve changing the expression host, using different vectors or promoters, altering the induction temperature, or co-expressing chaperone proteins.
In summary, protein expression and purification are multifaceted processes with numerous steps and decisions. Successful protein production and isolation often require a combination of standard protocols and empirical optimization.
Antibody Engineering Research Support Services
At Zebra BioSolutions, we leverage state-of-the-art technologies and deep expertise to propel your antibody projects forward. From early-stage research to late-phase optimization, our team is committed to transforming your vision into therapeutic and diagnostic realities.
Antibody Discovery and Generation:
Phage Display Libraries: Harnessing diverse phage display libraries to identify high-affinity antibodies against challenging targets.
o its native conformation.
Hybridoma Technology: Time-tested methods to produce monoclonal antibodies from immunized animals.
Single B-cell Cloning: Direct isolation of antigen-specific B-cells to obtain native antibodies.
Humanization & Chimerization:
CDR Grafting: Seamlessly transferring complementarity-determining regions to human antibody frameworks, maintaining affinity while reducing immunogenicity.
Framework Substitution: Modifying murine antibodies to replicate the characteristics of human antibodies, ideal for therapeutic applications.
Affinity Maturation:
Directed Evolution: Evolving antibodies in vitro for heightened target specificity and affinity.
Computational Modeling: Predictive modeling to identify potential improvement sites on antibodies, reducing experimental overhead.
Format Engineering:
Bispecific & Trispecific Antibodies: Crafting multi-specific antibodies to target multiple antigens or epitopes.
Antibody-Drug Conjugates (ADCs): Merging potent drugs with target-specific antibodies for precise delivery.
Fc Engineering: Modifying the constant region for improved stability, effector function, or extended half-life.
Functional Assays & Validation:
Biochemical Assays: ELISA, SPR, and BLI for rigorous assessment of antibody binding and kinetics.
Cell-Based Assays: Flow cytometry and cell-based neutralization assays to validate functional activity in physiologically relevant settings.
Scale-Up & Production:
Upstream Processing: Robust mammalian cell expression systems for high-yield antibody production.
Downstream Processing: Advanced purification techniques ensuring high-quality antibody preparations.
Analytical Characterization: Comprehensive analytics, from mass spectrometry to glycan profiling, ensuring product consistency and reliability.
Regulatory & Clinical Support:
Documentation: Assisting in the preparation of Investigational New Drug (IND) applications and regulatory dossiers.
Stability Studies: ICH-compliant stability studies to assess and ensure long-term antibody product integrity.